A urinary sediment examination
Abstract
A urinary sediment examination is an important type of non-invasive, repeatable morphological examination. It is necessary to accurately classify and measure urine components, such as epithelial cells, non-epithelial cells (blood cells), casts, salts/crystals, and microorganisms. The clinical significance of a urinary sediment examination is twofold. First, this examination is used to screen for the presence of a lesion in the kidney or urinary tract; second, it is used as a means to collect information on therapeutic and adverse effects of drugs administered to treat a confirmed lesion in the kidney or urinary tract. Pathological conditions are deduced not only from the results of a urinary sediment examination but also from a comprehensive evaluation of the results from various qualitative urinary examinations, such as urinary protein and occult blood tests, as well as biochemical (blood chemical) examinations. However, advances in diagnostic imaging and immunological examinations have allowed the current use of these methods for evaluating lesions in the kidney and urinary tract, and in consequence, the value of a urinary examination used as a screening test has increased further. Given these circumstances, we wished to conduct examinations with a clear understanding of their purpose; in other words, we hope to effectively conduct urine screening examinations, consider pathological conditions based on urinary findings, and observe and provide information useful to patients and for screening participants. In this part, we will explain the role of a urinary sediment examination and also the related technical methodology.
I What is Desirable in a Urinary Sediment Examination?
Urinary sediment examinations have played an important role in the diagnosis and assessment of therapeutic effects primarily in patients with urinary tract infections and other diseases of the kidney and urinary system (e.g., diabetic nephropathy or nephrotic syndrome). However, it is necessary to present value-added information to clinical parties in addition to classifying urinary sediment components and reporting calculations to more effectively use a clinical urinary sediment examination. Here “value-added information” refers to information pertinent to etiology. Pressures from medical cost-containment efforts and societal aging have increased the impractical nature of examinations that place large burdens on patients, such as renal biopsy and cystoscopic examinations; in this context, a painless urinary sediment examination has become essential.
However, morphological analysis (except for atypical cells and some other components) in the context of a routine urinary sediment examination has become an auxiliary diagnostic index for pathological conditions of the kidney and urinary tract since the advent of diagnostic imaging (e.g., ultrasonography and magnetic resonance imaging) and various immunological urine biomarkers. Therefore, we suggest a new developmental path for a urinary sediment examination. This path would fully incorporate the characteristics of urine that permit frequent, non-invasive examinations in an effort to understand, based on the detected components, why the components settled down and appeared in the urine, rather than merely performing a simple morphological component analysis.
II Basic Instructions
Urinary sediment examination results depend on urine sampling and urinary sediment sample preparation methods. Here we will present some examples from day-to-day operations that would require appropriate actions to be taken or that are encountered relatively frequently.
Urine types are distinguished by the time (e.g., morning urine or spot urine) and method (e.g., natural urine or catheter urine) of collection (see p.9 Table 1.1). As it is important to know the type of urine specimen that will be examined, an explicit description of the urine collection time and method should be encouraged.
Urine specimens are usually self-collected. To obtain suitable urine specimens, subjects should understand the requirements for a urinary sediment examination and receive guidance about appropriate urine collection methods. In particular, women must receive guidance regarding urine collection from an anatomical point of view, with an emphasis on wiping procedures to avoid contamination by components from the vulva [e.g., red blood cells (RBCs), white blood cells (WBCs), squamous epithelial cells, or bacteria].
However, contamination by irrelevant components may be unavoidable even after providing better urine collection guidance. Therefore, it is desirable to provide relevant comments when reporting such cases.
Examples of comments regarding suspected contamination by vulva-derived components
• The urine specimen is contaminated.
• Contamination of the urine specimen by components from the vulva is suspected.
• Contamination of the urinary sediment by components from the vulva (e.g., RBCs, WBCs, squamous epithelial cells, or bacteria) is indicated.
* In addition to commenting, it is necessary to discuss the need for the resubmission of a urine specimen with the attending physician.
The quantity of formed elements (i.e., sediment volume) clearly exceeds 0.2 mL in samples from subjects with conditions such as advanced hematuria and pyuria. It is desirable to provide a comment regarding such findings in the report.
Examples of comments when the sediment volume exceeds 0.2 mL
• The quantity of formed elements (sediment volume) exceeds 0.2 mL because of advanced hematuria.
• The quantity of formed elements (sediment volume) exceeds 0.2 mL because of advanced pyuria.
• A large amount of urinary sediment was obtained.
* Such specimens may require a microscopic examination and the reporting of formed elements (sediment elements) after mixing the sediment to the best possible homogeneity after centrifugation and removal of the supernatant with an appropriate procedure.
While the Japanese Committee for Clinical Laboratory Standards (JCCLS) uses per field units [/high power field (HPF) or /low power field (LPF)] to describe RBCs and WBCs, respectively, in a urinary sediment examination, guidelines from developed countries in Europe, as well as the United States [e.g., National Committee for Clinical Laboratory Standards (NCCLS)1), The European Confederation of Laboratory Medicine (ECLM)2)], recommend describing results in per μL units. The per μL expression, which is based on a counting chamber or uncentrifuged urine method, is also used in the efficacy evaluation criteria for urinary tract infection (UTI) drugs. Therefore, it is important to determine the volume (μL) corresponding to a single field of view during a sample preparation/microscopic examination according to the JCCLS examination method. However, urinary sediment preparation methods must still address the issues of components remaining in the urine supernatant during the centrifugation step or the adsorption of components to the tube wall. In theory, the following consideration is valid.
Area of field of view
= π × (number of field of view of the eyepiece lens/magnification factor of the objective lens × 1/2)2
Uncentrifuged urine equivalent volume per field of view (μL)
= area of field of view × urine concentration ratio × sediment load/area of the coverslip
At 20 fields of view, the areas of a single field of view are 3.14 mm2 for a low power field (LPF; objective lens: 10×) and 0.196 mm2 for a high power field (HPF; objective lens: 40×). Therefore, single fields of view correspond to the following amounts of uncentrifuged urine (μL):
LPF: 7.27 μL, HPF: 0.45 μL
According to the UTI Study Group, the following per μL expressions are based on the counting chamber method/uncentrifuged urine method:
0–9/μL 10–29/μL 30–99/μL ≥ 100/μL
III Staining Techniques
In principle, the specimens without staining is used for microscopic examinations of urinary sediments. Staining procedures may cause hemolysis and interfere with observations of the numbers and shapes of RBCs in urine. The color characteristics of sediment elements may also be lost. Therefore, it is important to use unstained specimens for observation.
However, the use of various suitable staining methods may be useful when urinary sediment elements must be confirmed and identified or differentiated from analogous components.
Basic staining solutions include the Sternheimer staining (S staining) and Sternheimer–Malbin staining (SM staining). When using these staining methods, an approximate 4:1 ratio of urinary sediment and staining solution is recommended while considering possible dilution errors related to the staining solution.
<Reagent>
Solution I: 2% Alcian blue 8GS in water
Solution II: 1.5% Pyronin B in water
Solutions I and II are filtered and mixed in a 2:1 ratio. The staining performance of this mixture remains stable for approximately 3 months if the mixture is stored in a cool and dark place.
<Staining procedure>
At the time of the microscopic examination, add a drop of the staining mixture to the sediment and mix.
<Staining behavior>
Red blood cells: unstained or pink/magenta
White blood cells: nucleus, blue; cytoplasm, pink/magenta
Epithelial cells: nucleus, blue; cytoplasm, pink/magenta (note: the stained cytoplasm of mucus-containing cells such as columnar epithelial cells and adenocarcinoma cells is bluish purple or dark magenta)
Macrophages: nucleus, blue; cytoplasm, bluish purple/dark magenta
Casts: hyaline casts, light blue/blue; granular casts and waxy casts, magenta

Figure 2.1
Sternheimer staining
<Reagent>
Solution I: Crystal violet 3.0 g
95% Ethanol 20.0 mL
Ammonium oxalate 0.8 g
Purified water 80.0 mL
Solution II: Safranin O 0.25 g
95% Ethanol 10.0 mL
Purified water 100.0 mL
Solutions I and II are mixed in a 3:97 ratio and filtered before use. A fresh mixture should be prepared every 3 months.
<Staining procedure>
At the time of the microscopic examination, add a drop of the staining mixture to the sediment and mix.
<Staining behavior>
Red blood cells: unstained or pale reddish purple
White blood cells: ① In dark cells, the nucleus is dark purple and cytoplasm is purple. ② In pale cells, the nucleus and cytoplasm are both unstained/light blue.
Epithelial cells: nucleus, purple/dark purple; cytoplasm, pink/purple
Casts: hyaline casts, pale red; granular casts, pale purple/dark purple granules; casts containing different cell elements exhibit unique staining patterns
<Reagent>
Dissolve 1.0–2.0 g of Sudan III in 100 mL of 70% ethanol with shaking, and allow this solution to rest in an airtight container in a 56–60°C incubator for 12 h, followed by storage at room temperature.
<Staining procedure>
Add 2–3 drops of the filtered solution to the sediment, allow the mixture to stand at room temperature (15–30°C) for 15–60 min, and evaluate the sediment via a microscopic examination. Sudan IV staining is also useful.
<Staining behavior>
Fat globules, fatty casts, oval fat bodies: yellowish red

Figure 2.2
Sudan III staining

Figure 2.3
Prescott–Brodie staining
<Reagent>
Solution I: 2,7-Diaminofluorene 300 mg
Phloxine B 130 mg
95% Ethanol 70 mL
Solution II: Sodium acetate·3H2O 11 g
0.5% Acetic acid 20 mL
Solution III: 3% Hydrogen peroxide water 1 mL
Solutions I, II, and III are mixed and filtered before use.
<Staining procedure>
At the time of the microscopic examination, add 5–10 drops of the staining solution to the sediment and mix well.
<Staining behavior>
Peroxidase-containing cells such as neutrophils, eosinophils, and monocytes: blue/blackish blue
Lymphocytes and other cells: red

Figure 2.4
Berlin blue staining
<Reagent>
Solution I: 2% Potassium ferrocyanide in water
Potassium ferrocyanide 2.0 g
Purified water 100 mL
Solution II: 1% Hydrochloric acid
Concentrated hydrochloric acid 1.0 mL
Purified water 100 mL
Store solutions I and II in a cool and dark place, and mix them in a 1:1 ratio immediately before use; the resulting clear, pale yellow solution should be used.
<Staining procedure>
Add 10 mL of the staining solution to 0.2 mL of sediment and mix. Allow the mixture to stand for 10–20 min, centrifuge, remove the supernatant, and subject the sediment elements to a microscopic examination.
<Staining behavior>
Hemosiderin granules: blue/indigo

Figure 2.5
Hansel staining
<Reagent>
Hansel staining solution:
Methylene blue 0.6 g
Eosin Y 0.2 g
Methanol 60 mL
Phosphate buffered saline (PBS) containing ethanol
Ethanol is added to PBS to a final concentration of 10%.
<Staining procedure>
Add 2 drops of the staining solution to 0.2 mL of the sediment; mix, and allow the mixture to stand for 5 min. Next, add 10 mL of 10% ethanol–PBS, mix/centrifuge, remove the supernatant, and subject the sediment elements to a microscopic examination.
<Staining behavior>
Eosinophil granule components: red

Figure 2.6
Lugol’s staining
<Reagent>
Lugol’s solution:
After dissolving 2 g of potassium iodide in approximately 10 mL of purified water, add and dissolve 1 g of iodine in this solution; subsequently, add purified water to a total volume of 300 mL (a freshly prepared reagent is preferable).
<Staining procedure>
Mix the sediment and staining solution in a 1:1 ratio, and subject the mixture to a microscopic examination.
<Staining behavior>
Epithelial cells: The cytoplasm of a glycogen-containing cell will be partly or entirely stained brownish red.
IV Procedures for Sample Preparation and Microscopic Examination
In the videos, methods to prepare urinary sediment specimens and adjust microscopes in urinary sediment examinations are explained by each. The points described are the most fundamental operations in the urinary sediment examination. They are considered as very important operational methods because they can have a great impact on the results. We aim to carefully observe these videos and acquire the skills for the urinary sediment examination.
1. Microscope adjustment method
1) Interpupillary distance and diopter adjustment
00:20
00:50
- IV
IV Download Video
2) Condenser adjustment (1)
00:05
00:49
- IV
3) Condenser adjustment (2)
00:02
00:40
- IV
4) Numerical aperture adjustment
00:00
00:38
- IV
2. Procedure for preparing urinary sediment specimen
1) Urine mixing/dispensing
00:00
00:54
- IV
2) Centrifuge selection
00:00
00:33
- IV
3) Supernatant removal
00:00
01:06
- IV
4) Preparing specimen (1)
00:00
00:43
- IV
5) Preparing specimen (2)
00:00
00:43
- IV
V Observational Methods of Urine Formed Elements
RBCs are important formed sediment elements that suggest the presence of hemorrhagic lesions in the kidney or urinary tract. An RBC is usually a pale yellow, disk-shaped cell, with a diameter of 6–8 μm and a dimple in the center. Its morphology varies depending on urine properties such as osmotic pressure and pH as well as the site of bleeding. RBCs have an atrophic appearance in urine with a high osmotic pressure or low pH, and a swollen, dehemoglobinized, or colorless ghost-like appearance in urine with a low osmotic pressure or high pH. In general, morphological changes due to urine properties are often homogeneous within a specimen. In healthy men and women, no more than four RBCs should be present per HPF.
*Morphology of RBCs in urine
Bleeding site-related morphological differences of RBCs in urine are important. In cases involving bleeding from the lower urinary tract (e.g., non-glomerular hematuria), RBCs may exhibit morphological changes, including disk-like (including confetti-like), spherical, swollen, and atrophic shapes, due to urine properties, such as osmotic pressure. Those shapes are nearly uniform and homogeneous in one specimen. These RBCs may exhibit slight variations in size and are rich in hemoglobin. In contrast, in cases of glomerular hematuria due to glomerular nephritis and similar conditions, RBCs exhibit various, non-uniform shapes and sizes, dehemoglobinized appearance, and frequent associations with various casts including RBC casts or proteinuria (Figure 2.7, 2.8).

Figure 2.7
Schematic diagrams of various isomorphic red blood cells (modified from “General Survey Technical Textbook” (2012)3) p. 58 Fig. 4-17)

Figure 2.8
Schematic diagrams of various dysmorphic red blood cells (modified from “General Survey Technical Textbook” (2012)3) p. 58 Fig. 4-16)
Regarding the reporting of RBC morphologies, isomorphic RBCs should be used to designate non-glomerular hematuria and dysmorphic RBCs should be used for presumed cases of glomerular hematuria in the comment. When reporting such cases, it is important to understand not only individual forms but also the overall sediment pattern; in addition, one should acknowledge that it is not always possible to classify hematuria (Figure 2.9, 2.10, 2.11).

Figure 2.9
Isomorphic red blood cells (40×, No staining)

Figure 2.10
Dysmorphic red blood cells (40×, No staining)

Figure 2.11
Elements often misidentified as red blood cells (40×, No staining)
RBCs cannot be consistently stained with S staining; some cells will stain red, whereas others exhibit little staining.
WBCs are important formed sediment elements; these cells are suggestive of the presence of inflammatory lesions, such as renal and UTIs. WBCs are usually spherical, with diameters of 10–15 μm; however, their morphology can vary depending on cell viability (viable cell or dead cell) and urine properties such as osmolality and pH. In general, WBCs tend to be atrophic at high osmotic pressures and become swollen at low osmotic pressures.
Although neutrophils account for the vast majority (approximately 95%) of WBCs found in urine, large numbers of lymphocytes, eosinophils, and monocytes can be found in association with various diseases and pathological conditions. The differential counts of these WBCs are highly clinically significant and should be reported as additional comments whenever they can be identified.
In healthy individuals, no more than four WBCs should be found per HPF.
Compared with other classes of WBCs, neutrophils have the highest levels of migration and phagocytic activity. The number of neutrophils in urine increases with urinary tract infection (e.g., cystitis, pyelonephritis, urethritis, or prostatitis). Viable and dead cells have different shapes. Viable cells exhibit various changes in shape, from spherical to rod-like, strip-like, and amoeba-like. The cell volume and density remain almost constant, and extended and expanded cells are very thin and unclear. In addition, the dropwise addition of 3% acetic acid solution to the sediment will enhance the clarity of viable WBC nuclei and facilitate the differentiation of these cells from small epithelial cells. Dead cells are readily affected by urine properties such as osmotic pressure and pH and are prone to exhibiting swollen and atrophic shapes.
Regarding S staining, viable cells are poorly stainable or appear virtually unstained, particularly immediately after staining. Cells can accordingly be classified as dark-stained, pale-stained, and glitter cells using S staining. Although glitter cells are reportedly observed at a high rate in pyelonephritis, these cells have low disease specificity. Often, atrophic dead cells exhibit good staining, whereas swollen dead cells are poorly stained. Prescott–Brodie staining (PB staining) yields blue to bluish black cell staining.
Compared to neutrophils, lymphocytes are smaller in size and have fewer granular components. These cells increase in number in situations such as chyluria, renal tuberculosis, renal transplant rejection, and chronic diseases. Lymphocytes are PB-stain negative.
Eosinophils are comparable in size to neutrophils and contain cytoplasmic eosinophilic granules. These cells increase in number in cases of interstitial nephritis, allergic cystitis, urolithiasis, and parasites. They are Hansel-stain positive.
Monocytes are larger than eosinophils and have unclear cytoplasm with various morphological changes. These cells increase in number in cases involving chronic UTI, prostate disease, glomerular disease, and treatment with anticancer drugs. (Figure 2.12, 2.13, 2.14, 2.15)

Figure 2.12
Neutrophils and lymphocytes

Figure 2.13
Neutrophils, eosinophils, and monocytes

Figure 2.14
Elements often misidentified as white blood cells

Figure 2.15
White blood cells and various staining methods (modified from “General Survey Technical Textbook” (2012)3) p. 59 Fig. 4-18)
Macrophages are phagocytic cells that emerge in association with inflammatory and infectious diseases of the kidney or urinary tract as well as pathological conditions such as enhanced tissue degradation.
The morphological characteristics of macrophages include a diameter of 20–100 μm and jagged or fuzzy and often unclear cytoplasmic margins. These cells have an indefinite circular shape. The cytoplasmic surface structure is faint and either cotton candy-like or homogeneous; in addition, these cells are highly permeable, and their nuclei can be readily observed without staining. Like other WBCs, macrophages are susceptible to changes in osmotic pressure and appear to be swollen in hypotonic urine; under these conditions, they look like sparsely distributed floating granular components such as fatty granules. In swollen macrophages, the marginal structure of the cytoplasm has a circular or near-circular shape. Phagocytosed dead cells (e.g., WBCs or RBCs), cellular fragments, crystals, and fatty granules may be observed in the cytoplasm. Unstained macrophages have a grayish-white color. They exhibit a good response to S staining; specifically, the nucleus is stained bluish purple, and the cytoplasm is usually stained magenta or bluish purple. Some kidney- and hourglass-shaped nuclei may be observed. Macrophages in a conglomerated form may require differentiation from columnar epithelial cells and adenocarcinoma cells; however, macrophages will not exhibit epithelium-like binding properties. In addition, some cells present with increased and enlarged or irregularly shaped nuclei; however, differentiation can be based on a small nucleus/cytoplasm (N/C) ratio, the absence of nucleolar enlargement, and/or no increase in chromatin amount.
In urinary sediment examinations, macrophages and monocytes are classified using a size threshold of 20 μm for the sake of convenience; cells measuring ≥20 μm are considered as macrophages, whereas those measuring <20 μm are considered as monocytes. (Figure 2.16)

Figure 2.16
Macrophages
Urine samples from women may be contaminated by endometrial cells at various times, such as during menstruation or after a gynecological examination. Endometrial cells comprise both epithelial and stromal cells, and both may be found in urine. It is generally difficult to distinguish these two types of endometrial cells when they are observed as conglomerates. Therefore, they are reported together as columnar epithelial cells (see p. 66 V.2.1.3 for more information).
If traffic between the urinary tract and abdominal cavity is generated because of conditions such as bladder rupture, mesothelial cells may be found in urine. Mesothelial cells comprise the serous membrane that covers body cavities such as thoracic and abdominal cavities. These cells have a thick cytoplasm with an often unclear margin structure. MG staining and Papanicolaou staining are useful for differentiation.
Epithelial cells that appear in urine can be classified according to the anatomy of the renal–urinary tract system into renal tubular epithelial cells, which line the lumen from the proximal tubule to the loop of Henle, distal tubule, collecting tubule, and renal papilla; urothelial cells, which are derived from the mucosa that lines the renal calyx and renal pelvis to the ureter, bladder, and internal urethral opening; columnar epithelial cells, which are derived from the mucosa of the membranous and spongy part of the male urethra and the mucosa of a part of the female urethra; and squamous epithelial cells, which are derived from the mucosa in the vicinity of the external urethral opening (Figure 2.17). These are referred to as basic epithelial cells. In addition to these basic cells, epithelial cells also include oval fat bodies and intracytoplasmic and intranuclear inclusion-bearing cells, which are classified based on cell morphological characteristics. In addition, human polyomavirus-infected cells and human papillomavirus-infected cells may appear.

Figure 2.17
Cells found in urine and points of differentiation
Regarding the histological classification of cells present in urine, no definitive findings allow the conclusive identification of any cell type, and it is difficult to differentiate various cells solely based on a single aspect. It is therefore important to understand several morphological characteristics of individual cells and thus make comprehensive judgments. In particular, findings such as cell size, cytoplasm margin and surface structures, and color are important, and unstained normal cells in urine can be differentiated to a substantial extent by focusing on these findings. However, staining methods can effectively differentiate cells that are atypical or otherwise difficult to identify. Among the staining methods, S staining is an excellent method that differentially stains the cytoplasm and nucleus and allows clear visualization of intranuclear structures. Most difficult-to-identify or atypical cells can be differentiated through the observation of both unstained and S-stained cells. However, if the cell origin is difficult to determine and the cell types cannot be specified, cells should be reported as unclassifiable, with an attached drawing or comments, to avoid expending more than a reasonable effort.
Renal tubular epithelial cells are frequently observed in urine samples from patients with renal parenchymal diseases, such as glomerular nephritis, nephrotic syndrome, renal sclerosis, lupus nephritis, and cystic kidney. In addition, these cells are frequently observed in association with non-renal diseases, including conditions that lead to renal ischemia or a decreased renal plasma flow (e.g., traumatic/surgical/obstetric hemorrhage, excessive diarrhea/vomiting, severe burns, severe hemolysis due to incompatible blood transfusion, advanced dehydration, or heart failure) and renal damage or allergic reactions caused by various chemicals and drugs (heavy metals such as mercury/lead/cadmium, organic solvents such as carbon tetrachloride/ethylene glycol, or pharmaceuticals such as salicylic acid/gentamicin/various anticancer agents). In addition, large numbers of renal tubular epithelial cells and various casts are found in urine samples from patients with diabetic nephropathy, hepatitis accompanied by jaundice, and various other conditions. As mentioned above, renal tubular epithelial cells are found in various pathological conditions/diseases; a small number of these cells are also found in urine samples from a minority of healthy individuals.
The morphological features of renal tubular epithelial cells most commonly encountered in routine practice include an approximate size of 10–35 μm, jagged or uneven saw-like cytoplasm margin structures, and irregular and granular cytoplasmic surface structure. The S stain yields the best staining quality, and cells are stained magenta. In addition, some cells contain spinous processes in the cytoplasm margin, cleaved spinous processes that appear similar to amoeba pseudopods (amoeba pseudopod-type), and prisms with strong spatial effects (prismoid type); these can be differentiated based on morphological characteristics. These renal tubular epithelial cells contain an eccentrically located, concentrated nucleus in common that is as large as an RBC. To differentiate renal tubular epithelial cells in actual urinary sediment microscopic examinations, it is important to first observe this type of cast-enclosed cell in detail and obtain a good understanding of its morphological features.
Renal tubular epithelial cells can exhibit a wide range of shapes, in association with their various location-dependent functions. In addition, these cells exhibit special forms and atypia under the influences of severe chronic renal failure and/or some drugs (e.g., anticancer agents/antibiotics) and may require differentiation from similar cells or casts and malignant cells.
• Circular and near-circular forms appear as clumps with primarily radial arrangements and have a clearly curved cytoplasm margin structure, fine mesh-like or homogeneous cytoplasmic surface structure, WBC-sized and eccentrically located nucleus, and occasionally visible nucleoli. Care should be exercised to differentiate these cells from adenocarcinoma cells. Renal tubular epithelial cells are distinguishable because they exhibit no increase in chromatin amount.
• Tadpole/snake and fiber forms appear as clumps in a bundle or radial arrangement, with a thin cytoplasm and apparently homogenous cytoplasmic surface structure. Although care should be taken to discriminate these cells from similarly shaped squamous carcinoma cells, no atypia (e.g., increased N/C ratio and chromatin amount) is observed.
• Pear/spindle forms are often attached to casts and have a square-shaped/multisided cytoplasm margin structure; differentiation from urothelial cells is necessary. This differentiation is possible because of the thin cytoplasm, homogenous cytoplasmic surface structure, and unclear, wrinkled, and bent cytoplasm margin structure.
• Granular and vacuolar denatured cast forms require differentiation from their respective casts; however, close observation reveals single cells containing a nuclei with a size similar to that of one or two WBC. Cells containing brown hemosiderin granules are occasionally found in urine samples from patients with hemolytic diseases (e.g., paroxysmal nocturnal hemoglobinuria). The mechanism of urine hemosiderin production suggests that these cells are renal tubular epithelial cells.

Figure 2.18
Characteristics of renal tubular epithelial cells (40×)

Figure 2.19
Characteristics of renal tubular epithelial cells (40×)

Figure 2.20
Characteristics of renal tubular epithelial cells (40×)
*Novel round cells (round type renal tubular epithelial cells)
These cells are small and have a circular shape. The cell color is grayish white, and the cytoplasm surface structure is homogeneous and relatively thick; however, it is thinner than deep layer squamous epithelial cells. The margin structure is curved. In S staining, the cells are stained poorly or pale pink. These cells have the potential to differentiate and are thought to mostly differentiate into renal tubular epithelial cells. They are discharged into the urine when the renal function is severely impaired.
Clinically, urothelial epithelial cells are found in association with inflammation, calculosis, and catheter-related mechanical injuries in areas ranging from the renal calyx/pelvis to the internal urethral opening; related conditions include cystitis, pyelonephritis, and ureteral calculi. Histologically, urothelial cells form a 1–6-layer, multirow epithelial layer and are often observed in a conglomerated form. These cells are divided into surface layer and middle layer–deep layer cells.
These cells range in size from 60 to 150 μm and have an angular cytoplasmic margin structure and ridge-like shape and often present as a multisided form. The cell color is yellowish; the cytoplasmic surface structure is rough, and cells generally have two or three nuclei. The cells are well stained with S staining to a magenta color.
These cells range in size from 15 to 60 μm and have an angular cytoplasmic margin structure, a spindle or pear shape, or a multisided form. In addition, some newer cells exhibit a tailed structure. The cytoplasm is thick, and the cytoplasmic surface structure is rough. Similar to surface layer cells, unstained cells are yellowish, and S staining yields a strong magenta color. Because nuclear sizes are comparable among surface layer, middle layer, and deep layer cells, deep layer cells appear to have a higher N/C ratio, and care should be taken when differentiating these cells from malignant cells. (Figure 2.21, 2.22)

Figure 2.21
Characteristics of urothelial cells (40×, No staining)

Figure 2.22
Characteristics of urothelial cells (40×, S staining)
Columnar epithelial cells are observed in conditions such as urethritis, mechanical injury to the urinary tract due to catheter insertion, or after ileal conduit urinary tract diversion. In addition, columnar epithelial cells derived from the prostate and seminal gland may be observed in samples from men with prostatitis, prostatic hyperplasia, or vesiculitis or after prostate massage. Because of anatomical structural characteristics, urine samples from women may be contaminated by columnar epithelial cells from the uterine endometrium. In particular, caution should be exercised when analyzing urine samples collected during menstruation or after the mechanical scraping of the uterus for cytological testing because a large number of uterus-derived columnar epithelial cells may be observed alone or in conglomerations with RBCs, WBCs, or other cells. When columnar epithelial cells are observed and their origin can be deduced, comments such as “likely to be prostate columnar epithelial cells” should be added.
Morphologically, columnar epithelial cells are generally small in size (approximately 15–30 μm) and have an angular cytoplasmic margin structure. Therefore, caution is required when differentiating these cells from deep layer urothelial cells. The cell shape is usually cylindrical, rectangular, or teardrop-like with a flat end, and some new or well-preserved columnar epithelial cells have a pilus on the flat end, which is important for differentiation. In addition, these generally do not vary in size, are thinner than deep layer urothelial cells, and have a homogeneous or pale mesh-like cytoplasmic surface structure. Several small granules are often present between the flat portion of the cytoplasm and the nucleus, and cells appear grayish white without staining. With S staining, cells are well stained and appear magenta, bluish purple, or dark magenta in color. (Figure 2.23)

Figure 2.23
Characteristics of columnar epithelial cells
Squamous epithelial cells are commonly found in cases of urethritis due to Trichomonas vaginalis and bacterial infection, urethral calculi, mechanical injuries due to catheter insertion, and during estrogen treatment for prostate cancer. In addition, urine samples from women are likely to be contaminated by squamous epithelial cells derived from the vulva and vagina, along with RBCs, WBCs, and bacteria, even if a subject’s urinary tract is free of abnormalities. Therefore, guidance regarding hygiene, such as instructions for collecting midstream urine after opening and cleaning the labia minora, should be given at the time of urine collection. Histologically, cells are arranged in multiple layers parallel to the basement membrane and are composed of middle layer–deep layer cells and surface layer cells. In samples from women, cell shape may vary depending on the sexual cycle. Abnormal shapes, enlargement, and multinucleation can occur during estrogen treatment and radiation therapy, and caution should be exercised when differentiating these cells from malignant cells.
These cells range in size from 60 to 100 μm and have a mainly irregular shape and remarkably thin cytoplasm. Although the cytoplasmic surface structure is homogeneous, the margin is often twisted, bent, or wrinkled. With S staining, cells are well stained to a magenta color.
These cells range in size from 20 to 70 μm and have a rounded cytoplasmic margin structure and circular or near-circular cell shape. The cytoplasm is thick, and cells acquire a spherical shape as they move closer to the deep layer. The cytoplasmic surface structure is homogeneous, but cells may exhibit depression-like or pleated structures where a part of the cell appears invaginated. Unstained cells appear shiny gray or green in color. Cells stain poorly with S staining and often appear as a pale pink color. This phenomenon could be attributed to the high glycogen content of cells in this layer; in contrast, these cells are brown to brownish red in color after Lugol’s staining. (Figure 2.24)

Figure 2.24
Characteristics of squamous epithelial cells
*Podocytes
Podocytes are small cells and have a circular shape. The cell is grayish white, and the cytoplasm surface structure is homogeneous or fine granular and relatively thick; however, it is thinner than deep layer squamous epithelial cells. The margin structure is curved. In S staining, the cells are stained poorly or pale pink. They are discharged into the urine in patients with highly active IgA nephropathy or focal glomerulosclerosis.
These are fat granule cells that appear in association with renal impairment and are derived from either renal tubular epithelial cells or macrophages. Fat granule cells are not distinguished according to their origins and are collectively referred to as oval fat bodies. These cells are found at particularly high numbers in urine samples from patients with severe nephrotic syndrome and are included among diagnostic criteria for this disease. These cells are also found in urine samples from patients with severe diabetic nephropathy, Fabry disease, and Alport syndrome.
Morphologically, these cells range in size from 10 to 40 μm and have a circular, near-circular, or irregular shape; in addition, if the fat granule content is high, fat granules may extend from the cell margin in droplet forms, and cells may exhibit a pseudorosette-like shape. Unstained small fat granules have a shiny black or bronze color, whereas large fat granules are shiny yellowish. Fat granules do not respond to S staining, and stained cells appear similar to unstained cells.
It is often difficult to identify the origins of oval fat bodies and other fat granule-containing cells solely based on their morphological features. However, it is possible to distinguish cells from other cell types according to the background and other factors. Cells can be identified as oval fat bodies when similar fat granule cells are also observed in casts or when fatty casts are observed. In addition, corresponding urinary protein levels are often strongly positive.
Fat granule-containing cells other than oval fat bodies in urine are mostly prostate-derived macrophages; accordingly, it is important to confirm the subject’s sex, age, and type of urine (e.g., prostate massage urine). In addition, neutrophils that appear in the background often contain fat granules.
Sudan III staining and observation under a polarizing microscope are widely used to confirm fat granules. The appearance of successful Sudan III staining varies depending on the type of fat. In general, cholesterol esters and fatty acids are stained yellowish red, cholesterols are stained yellowish red-orange, triglycerides are stained red, and phospholipids and glycolipids are stained pale red. Meanwhile, when fat granules are observed under a polarizing microscope, cholesterol esters and phospholipids exhibit a characteristic multirefractory polarized appearance called the Maltese cross. However, care must be taken because triglycerides and fatty acids do not exhibit these multirefractory polarized appearances. (Figure 2.25)

Figure 2.25
Characteristics of oval fat bodies (fatty casts)
Although associations of measles, rubella, mumps, influenza, and other RNA viral infections with intracytoplasmic inclusion-bearing cells have been previously implicated, these associations have not been proven. Rather, because cells of this type are often found in urine samples from patients with cystitis or pyelonephritis, urinary tract diversion, and renal drug poisoning, they are considered as degenerated cells that appear at the time of non-specific inflammation. In addition, cells bearing inclusion bodies are remarkably degraded or degenerated, and the origins of these cells are often difficult to determine solely based on morphological characteristics. Therefore, they are generally reported as intracytoplasmic inclusion-bearing cells.
Morphologically, intracytoplasmic inclusion-bearing cells range in size from 15 to 100 μm and exhibit various shapes, including circular, near-circular, irregular, and multisided. The cytoplasmic surface structure also varies and includes homogeneous and granular forms, and the cytoplasm contains inclusion bodies in various shapes (e.g., circular, near-circular, doughnut-like, or horseshoe-like). Unstained inclusion bodies are similar in color to the cytoplasm, but they appear darker and slightly glossy. With S staining, inclusion bodies generally appear as a similar but darker color to that of the cytoplasm. (Figure 2.26)
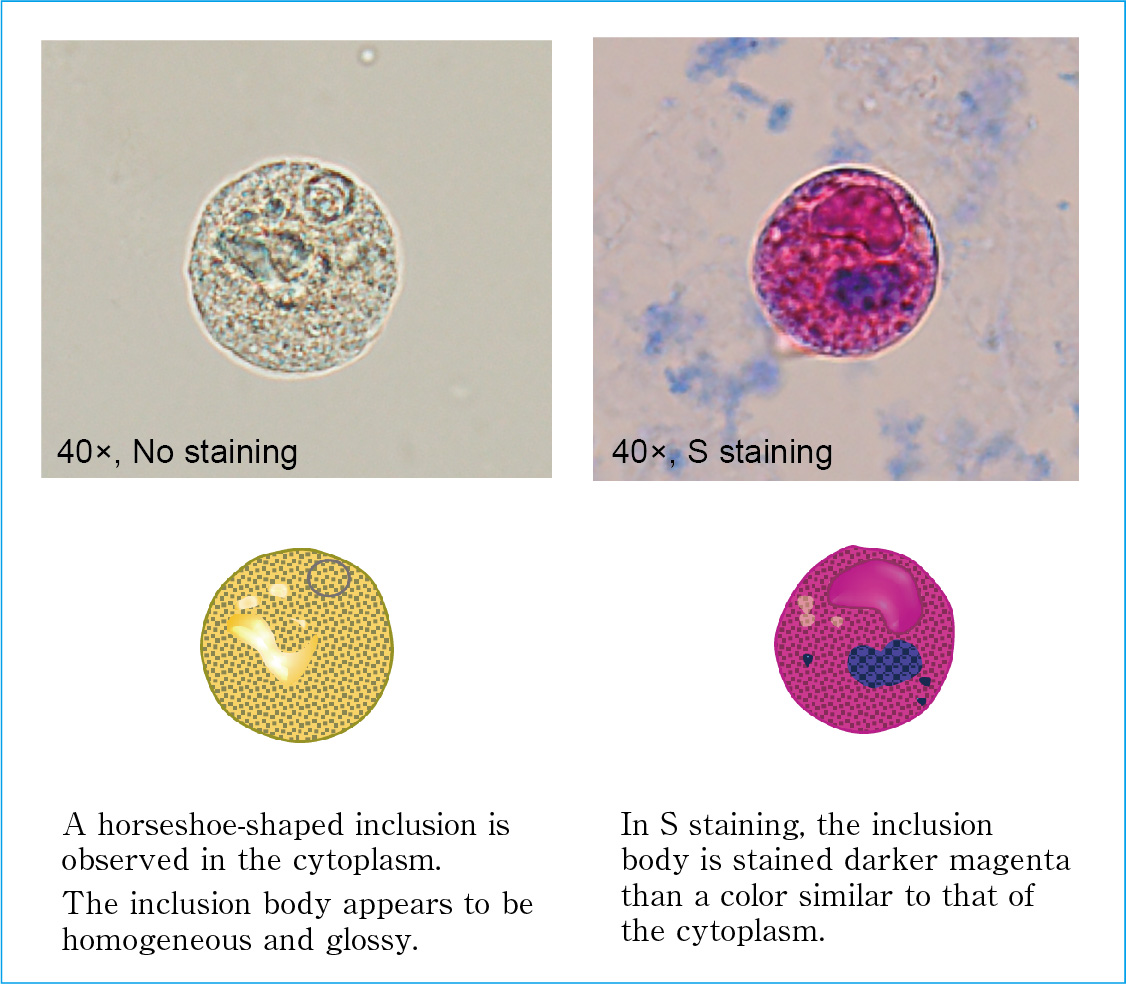
Figure 2.26
Characteristics of intracytoplasmic inclusion-bearing cells
Cells of this type are detected in urine samples from patients infected by DNA viruses such as herpesvirus and cytomegalovirus. Like intracytoplasmic inclusion-bearing cells, these cells are remarkably degraded or degenerated, and their origins are often difficult to determine solely based on morphological characteristics.
Morphologically, intranuclear inclusion-bearing cells range in size from 15 to 100 μm, with rare exceptions where cells are 200 μm or larger, and they primarily exhibit a circular or near-circular shape. Characteristic changes are observed in the nucleus; irregularly shaped amorphous inclusion bodies are formed in the nucleus, and chromatins aggregate at the nuclear contour. In addition, multinucleated giant cells with ground glass-like nuclei that may exhibit a molding pattern are sometimes observed. The colors of unstained and S-stained inclusion bodies are similar to those of intracytoplasmic inclusion-bearing cells. Multinuclear and mononuclear cells are considered to be herpesvirus- and cytomegalovirus-infected cells, respectively. When the virus is not identified using special staining or other techniques, cells are reported as suspected herpesvirus- or cytomegalovirus-infected cells. (Figure 2.27)

Figure 2.27
Characteristics of intranuclear inclusion-bearing cells
Human polyomavirus-infected cells appear with an increased N/C ratio and a ground glass-like nucleus. These cells are thought to be derived from urothelial cells and renal tubular epithelial cells based on their morphological features and occurrence patterns. When the virus is not identified using special staining, these cells are reported as suspected human polyomavirus-infected cells.
Human papillomavirus-infected cells are characterized by an extensively vacuolated cytoplasm around the nuclei of squamous epithelial cells. These cells are referred to as koilocytes and may be associated with atypia, such as an increased and enlarged nucleus and intensive nuclear staining. When the virus is not identified using special staining, cells are reported as suspected human papillomavirus-infected cells. (Figure 2.28)

Figure 2.28
Characteristics of virus-infected cells
Cells considered atypical in a urinary sediment examination are malignant or suspected malignant cells. Although the morphological characteristics of malignant cells differ according to histological type, nuclei generally exhibit many characteristics and atypia, such as enlarged nuclei, increased chromatin amount, irregular nuclear shape, and enlarged nucleoli relative to corresponding structures in normal cells. However, cells that exhibit atypia, or atypical cells, do not exclusively appear in malignant lesions but may also be observed in conjunction with benign lesions (e.g., inflammation, calculosis, or virus infection) or in response to chemical or physical agents (e.g., radiation or therapeutic agents). Accordingly, caution is required.
Atypical cells cannot be classified according to a single finding, and it is important to make comprehensive judgements based on detailed observations of cells from various angles, the range of individual cells that appear throughout the specimen, and other factors. In addition, a good understanding of the morphological characteristics of normal cells in the kidney/urinary tract system that may appear in urine is fundamental when attempting to detect malignant cells during a urinary sediment examination (Figure 2.29).

Figure 2.29
Characteristics of atypical cells
In urine, most malignant cells are urothelial cancer cells; squamous cancer and adenocarcinoma cells are rarely observed. A good understanding of the morphological characteristics of these cells is needed to predict the histological types of malignant cells.
Atypical cells found during a urinary sediment examination should be reported as follows to avoid confusion of clinical parties and provide additional useful information.
• Cells considered as atypical cells in urinary sediment examinations shall be reported as “malignant cells” or “suspected malignant cells.” In addition, cells for which possible malignancy cannot be ruled out should also be reported as atypical cells, even if atypia is weak. This reduces the risk that malignant cells will be overlooked and is important for a urinary sediment examination wherein the highest priority is placed on sensitivity.
• When actual atypical cells are to be reported, comments should be added always to the report, rather than simply “atypical cells (+)” or “suspected atypical cells.” Importantly, the comments should report the histological type, possible pathological conditions, and other relevant parameters in a manner that is easy to understand. Therefore, it is desirable to report atypical cells as specifically as possible as demonstrated in the following examples: atypical cells (suspected urothelial cancer), atypical cells (suspected adenocarcinoma, suspected invasion of colon cancer), and atypical cells (suspected squamous cell carcinoma, suggesting contamination by cervical cancer). Even if the histological type is unknown, a comment suggesting the presence of malignant cells, such as “atypical cells (malignancy suspected, histological type unknown),” should always be added.
• When describing atypical cells for which the possibility of malignancy cannot be ruled out, despite weak atypia, the histological type of observed atypical cells should be reported whenever possible as demonstrated by the following examples: atypical cells (difficult to determine benign/malignant, derived from urothelial cells), atypical cells (difficult to determine benign/malignant, derived from squamous epithelial cells), atypical cells (difficult to determine benign/malignant, histological type unknown).
• Cells with atypia that appear to be benign should be reported as the original histological type (e.g., urothelial cells, squamous epithelial cells) rather than atypical cells, and comments should be included as needed. In addition, among cells with atypia that appear benign, those for which the histological type cannot easily be determined should be reported as unclassifiable cells, with comments as needed.
• Cells with no atypia and an unknown histological type should be reported as unclassifiable cells.
Urothelial cancer originates in the urothelial layer, which extends from the renal calyx/pelvis to the internal urethral opening. Urothelial carcinoma emerges as a lesion of isolated/scattered or conglomerate cells; cell–cell junctions weaken, and cells tend to be more scattered as the histological level of atypia increases. In addition, cell cannibalism is an important finding as this strongly suggests the presence of a malignant tumor even if the nuclear atypia is weak. Urothelial carcinoma cells exhibit circular, near-circular, pear-like, angular, and other shapes. The angularity of the cytoplasm margin structure is an important finding that suggests a urothelial cell origin. Nuclei are enlarged and tend to be eccentric with increasing grades. In addition, the nucleus may occasionally appear to protrude from the cytoplasm. Nuclei form irregular shapes (e.g., near-circular, angulated/cleaved) and may exhibit three-dimensional irregularities (i.e., nuclear shape changes when the microscope focus is changed). The amount of chromatin, which appears in a coarse granular form, is increased, although these elements do not necessarily exhibit hyperchromatic S staining. Therefore, the possibility of pale nuclear staining should be noted during microscopic examinations. Regarding secondary findings, karyopyknotic cells that likely derive from urothelial carcinoma, intracytoplasmic inclusion-bearing cells, and cells containing fat granules are often observed in the background. If these cells are present, it is important to observe them carefully while considering the possible presence of urothelial carcinoma.
Primary adenocarcinomas of the renal/urinary system include renal cell carcinoma, prostate cancer, primary adenocarcinoma of the bladder (e.g., urachal cancer), and adenocarcinoma arising from metaplasia of the urothelium or of a urothelial carcinoma. In addition, cells from adenocarcinomas such as rectal cancer, ovarian cancer, and breast cancer may appear in urine in some cases involving invasion of or metastasis to the renal–urinary tract system. In well-differentiated cancers, cylindrical and near-circular cancer cells appear as corollaceous, palisading, or papillary cell clusters. Their cytoplasm is lighter than that of urothelial carcinoma cells and may contain mucus-like vacuoles. Nuclei are near-circular in shape and eccentric, and chromatins are present at increased amounts and in a fine granular form. In addition, adenocarcinoma cells often contain markedly large and very noticeable nucleoli.
Primary squamous cell carcinomas of the renal and urinary tract include squamous cell carcinomas arising from metaplasias of the urothelium or urothelial cancer and those arising from the squamous epithelial layer of the external urethral opening. In addition, these cells are observed in urine when cervical cancer directly invades the bladder or when cancer cells contaminate urine through the vulva. Approximately 80% of squamous cell cancer cells detected in the urinary sediment of women are said to be derived from cervical cancer. Therefore, when squamous carcinoma cells are detected in samples from women, it is important to take into account the possibility of cancer not only in urological organs but also in the cervix in the examination.
Squamous cell carcinoma cells have irregular shapes (e.g., snake-like, tadpole-like, or fibrous cell-like), generally with large nuclei and a noticeable increase in the amount of chromatin. Circular and near-circular cancer cells exhibit a thickened cytoplasm, concentric layered structure, and centrally located nucleus. In addition, anuclear cancer cells are found in squamous cell carcinomas with strong keratinizing tendencies, and caution should be exercised to not overlook these cells.
Small cell carcinoma cells in urine exhibit morphological characteristics similar to those of small cell lung cancer cells; these cells are similar in size to WBCs and may be present in isolated, scattered, or conglomerated forms. In addition, the characteristic appearances of a Kimekomi-like or rosette-like arrangement are occasionally seen. Because these cells mostly contain a reduced amount of cytoplasm, the observed cells may have bare nuclei. Chromatin, which increases in amount, exhibits a fine granular appearance. Although urothelial cancer and prostate cancer cells may also appear similar in size to WBCs, differentiation from those cells can be achieved based on the above characteristics. Neuroendocrine granules are often visible in the cytoplasm via electron microscopy and can be confirmed by immunohistochemical staining to prove the presence of neuroendocrine markers such as neuron-specific enolase, chromogranin A, and synaptophysin.
Malignant epithelial cells, such as undifferentiated carcinomas, choriocarcinomas, and carcinoids, are rarely observed.
Malignant lymphoma cells may be found in urine as a result of primary or metastatic/infiltrating lesions present in lymph tissues in the bladder and urinary tract. These cells are often approximately two-fold larger than WBCs and appear as isolated, scattered circular cells. The N/C ratio is high; the increased amounts of chromatin appear as coarse granules, and nucleoli are enlarged. These cells are similar to small cell carcinoma cells in terms of the high N/C ratio, but they can be differentiated from those cells based on the isolated/scattered appearance and distinct chromatin findings.
Leukemia cells are mainly found in urine samples from patients in whom these cells are circulating in peripheral blood. These cells, along with hematuria, are more likely to be observed in leukemia patients with increased bleeding diathesis. Differentiation from malignant lymphoma cells is difficult and is achieved by considering clinical information.
Other non-epithelial malignant cells include malignant melanoma cells, leiomyosarcoma cells, fibrosarcoma cells, and rhabdomyosarcoma cells.
Casts are elements formed using the renal tubule lumen as a template and are therefore mainly cylindrical in shape. The matrix component is a gelatinous solidified precipitate composed of Tamm–Horsfall mucoprotein, which is secreted by renal tubular epithelial cells, and a small amount of plasma proteins. Hyaline casts comprise only this matrix component, whereas various other types of casts are formed by the inclusion and subsequent disruption and degeneration of blood cells, renal tubular epithelial cells, and other elements.
The emergence of casts indicates that the renal tubule lumen was temporarily occluded, followed by the reestablishment of urine flow. Kidney and renal tubular pathologies and impairment extent can be understood according to the type, number, and shape of the observed casts.
The Lippman classification system and its derivatives have been previously used to classify casts. However, because these classification systems are not entirely suitable for use in routine examinations, a new, simplified classification method that incorporates clinically significant considerations has been presented in Japan as part of JCCLS GP1-P3 (2000). In this book, we follow this new method.
The best effort should be made to fully differentiate the following types casts as these can be differentiated using routine urinary sediment microscopic examination methods, and their clinical relevance has been elucidated. (Figure 2.30, 2.31)

Figure 2.30
Mechanism of cast formation (modified from “General Survey Technical Textbook” (2012)3) p. 71 Fig. 4-37)

Figure 2.31
Degenerative process of casts (modified from “General Survey Technical Textbook” (2012)3) p. 71 Fig. 4-38)
Hyaline casts represent a substrate for various types of casts. These typically have a cylindrical shape with rounded ends and parallel long sides; however, shape variations include inflected, S-shaped, and cleaved forms. Hyaline casts also include a type of formed element with a narrowed end, which has been previously described as a cylindroid. The morphological characteristics of these casts range from homogeneous and non-structured to wrinkled and streaky. A variety of cast types may be observed, including single-component casts with no other contents and those enclosing small amounts of various components (up to two blood cells, renal tubular epithelial cells, or fat granules; granular components accounting for less than one-third of the total cast).
Unstained hyaline casts are faint and easily overlooked; therefore, care should be taken. S staining renders these casts from pale to dark blue; however, differentiation from mucus threads may be required.
Hyaline casts may also be found in urine from healthy individuals, particularly those who experience frequent dehydration associated with intense exercise. However, persistent detection in a healthy individual should be considered as clinical information. In addition, these casts may be observed in patients with kidney disease-related proteinuria, systemic blood flow disorders, and other conditions.
Epithelial casts enclose renal tubular epithelial cells within the substrate. These casts exist in various states, ranging from a state in which three epithelial cells are enclosed to a state in which a cast is very densely packed with epithelial cells. Epithelial casts also include casts to which epithelial cells are attached. These casts are primarily observed in patients with kidney/renal tubular disorders. Differentiation is required from WBC casts when specimens are unstained and from granular casts when specimens are denatured.
After S staining, the cytoplasm of renal tubular epithelial cells associated with casts often exhibits a red-to-magenta with blue nuclei, although some cells are anuclear.
Granular casts are casts in which granular components enclosed within the substrate account for at least one-third of the total components. These granular components mainly comprise degenerated renal tubular epithelial cells but may also include other types of degenerated cells such as RBCs and WBCs. In addition, some granule components may appear to be derived from plasma proteins. Granules may be coarse or fine. In either case, casts are classified as granular cast. When a granular cast encloses three or more cellular components or is undergoing transition from a cellular cast to a granular cast, both cellular cast and granular cast should be reported.
Granular casts are strongly associated with decreased kidney function related with many renal diseases and also indicate damage to the renal parenchyma.
After S staining, granular casts appear as a pale to dark magenta or dark bluish purple.
Waxy casts were so named because a part of or the entire cast has a homogeneous and non-structured wax-like appearance. These casts are thought to be formed by the advanced degeneration of cellular or granular components in casts as a result of the prolonged blockage of the renal tubule lumen or homogeneous agglomeration of plasma proteins. Although cleavages are often observed, waxy casts exhibit a variety of shapes, including S-shaped, inflected, and puffball-like/salmon roe-like. Many waxy casts are thick, shiny, and highly refractive. Waxy casts have a clear contour and can be easily distinguished from hyaline casts. When three or more cellular components are enclosed in a waxy cast, both waxy cast and cellular cast should be reported. A granular cast that is transitioning to a waxy cast or appears in a mixed form should be classified as a waxy cast.
Waxy casts are primarily observed in patients with serious renal diseases such as nephrotic syndrome, renal failure, and terminal-stage nephritis.
After S staining, waxy casts appear pale to dark magenta or dark bluish purple.
Fatty casts enclose fat granules and oval fat bodies within the substrate. These casts appear in many forms, ranging from the enclosure of three fat granules to a cast very densely packed with fat granules. Because many oval fat bodies contain at least three fat granules, a cast that encloses even one oval fat body is also classified as a fatty cast. Fatty casts are observed frequently in patients with nephrotic syndrome.
Fat granules do not respond to S staining but exhibit an orange-red to red in response to Sudan III (IV) staining. In addition, these casts can be confirmed by the appearance of polarized images, such as Maltese crosses, under a polarizing microscope (see p. 71 V. 2. 2. 1).
RBC casts incorporate RBCs into the substrate. These casts take various forms, including the enclosure of three RBCs and a structure so densely packed with RBCs that no extra space is present. In some casts, RBCs exhibit the standard disk-shaped or spherical hemoglobin-containing morphology; however, these cells exist in a dehemoglobin status in majority of the casts. Unstained RBCs in casts appear a reddish brown if they have undergone granulation or waxy degeneration, and highly degenerated or degraded RBCs often remain outside the contour. However, care must be taken because all reddish brown-colored casts are not necessarily derived from RBCs.
RBC casts indicate bleeding in the nephron and are clinically observed in urine samples from patients with diseases accompanied by renal bleeding such as IgA nephropathy, purpura nephritis, acute glomerulonephritis, membranoproliferative nephritis, lupus nephritis, and anti-neutrophil cytoplasmic autoantibody (ANCA)-associated nephritis.
WBC casts are characterized by the enclosure of WBCs in the substrate. These casts appear when infection or inflammatory disease of the nephron is present. Differentiation from epithelial casts may be necessary when the specimen is unstained or from granular casts when WBCs are degraded. The enclosed WBCs are mainly neutrophils, although lymphocytes and monocytes may be primarily present in some cases, depending on the clinical condition.
WBCs are easy to differentiate because the nuclei exhibit positive S staining, whereas the cytoplasm is often poorly stained. This characteristic can be used to differentiate WBCs from well-stained renal tubular epithelial cells.
WBC casts that mainly contain neutrophils are found in patients with active-phase acute glomerulonephritis, pyelonephritis, and similar disease, whereas casts containing lymphocytes and monocytes are found in patients with chronic diseases. WBC casts containing eosinophils may be found in patients with interstitial nephritis.
Vacuolar denatured casts are casts containing vacuoles of various sizes. Some casts are entirely filled with vacuoles, whereas others are partially vacuolated granular casts and waxy casts. These casts are often seen in severe cases of diabetic nephropathy and are often accompanied by severe proteinuria or reduced renal function.
Following S staining, these casts mainly appear magenta, although some exhibit a bluish purple. They are believed to be derived from vacuolated renal tubular epithelial cells and lysed fibrin casts, which are described below.
Salt/crystal casts enclose amorphous salts (phosphates and urates), calcium oxalate crystals, or drug crystals. These are thought to result from crystallization in the renal tubule lumen and obstruction, and they are useful for identifying tubulointerstitial involvement. In some cases, wide casts that expand the renal tubule lumen are formed; these are accompanied by fibrous or circular/near-circular renal tubular epithelial cells inside or outside the casts.
A macrophage cast is a cast with three or more macrophages attached or enclosed within it. Morphological features of macrophages can be observed in the cast. When unstained, viable cells are gray or grayish white, the cytoplasm surface structure is cotton candy-like, and the margin structure is an unclear saw-like shape. Dead cells are yellowish and often have a circular or near-circular shape. In S staining, dead cells generally show the cytoplasm stained in bluish purple or deep reddish purple, whereas viable cells are poorly stained.
In addition, macrophages are often observed to contain fat granules. However, if the macrophage in the cast contains three or more fat granules, it is considered as an oval fat body and the cast classified as a fatty cast. Macrophage casts are found in association with active nephrotic syndrome, advanced renal tubular damage, renal failure, myeloma of the kidney, and some other pathological conditions.
Fibrin casts are packed with fibers and S-stain negative. However, the fibrous structure can be sufficiently confirmed without staining. Still, verification that the cast substrate is unstainable is preferred as fibers in some casts are fused and homogeneous.
Some fibrin casts stain pale pink or pale blue in response to S staining; however, only those that can be clearly distinguished from other casts should be considered as fibrin casts.
These casts are often observed in patients with diabetic nephropathy and high-level background proteinuria and often simultaneously appear with or slightly earlier than vacuolar denatured casts.
(Figure 2.32)

Figure 2.32
Discrimination criteria for casts (modified from “General Survey Technical Textbook” (2012)3) p. 73 Fig. 4-39)
Hemosiderin casts appear as yellow- to brown-colored granular casts in unstained specimens. They can be identified as blue elements using Berlin blue staining.
These casts are observed in paroxysmal nocturnal hemoglobinuria, intravascular red cell fragmentation syndrome, and other hemolytic diseases. Hemosiderin granules and hemosiderin-containing cells (i.e., renal tubular epithelial cells) are often simultaneously observed.
Like hemoglobin casts, myoglobin casts appear as reddish brown-colored waxy or granular casts. Immunochemical methods are required for verification. These casts are found in patients with myoglobinuria associated with rhabdomyolysis, crush syndrome, and similar conditions.
Bence Jones protein (BJP) casts are observed in urine specimens from patients with BJP-positive myeloma and often appear as puffball-like/salmon roe-like waxy casts. The methods used to confirm BJP casts include fluorescent antibody staining with an antibody specific for the immunoglobulin L chain.
*Confirmatory tests such as Berlin blue staining and immunostaining are always required for hemosiderin casts/myoglobin casts/BJP casts. If confirmation cannot be achieved, casts should be differentiated as waxy or granular casts depending on substrate properties, and add a comment such as suspected hemosiderin casts, suspected myoglobin casts, or suspected BJP casts as appropriate.
Microorganisms/parasites found in urinary sediments include bacteria, fungi, protozoa, and helminths.
Bacteria can be classified as bacilli and cocci. Bacilli are relatively easy to confirm via microscopic examinations with ×400 magnification, whereas cocci are generally difficult to differentiate/confirm. The determination of bacteria in urine is essential for the diagnosis of UTIs such as pyelonephritis and cystitis. However, the presence of resident bacteria in the urethra render it impossible to completely eliminate contamination, even if midstream urine collection is strictly conducted, unless urine specimens are collected via direct bladder puncture. Furthermore, when an inappropriate urine collection method is used, urine samples are contaminated by the abundant bacteria that reside around the urethral opening or in the vulva. Urine samples from women can be contaminated by a large number of lactobacilli from the vagina. In addition, deformed bacteria with elongated bodies may be observed in samples from patients using antimicrobials (cell wall synthesis inhibitors).
In general, simple UTIs are mostly caused by infection with a single bacterium; the etiological agent is Escherichia coli in the vast majority of cases, although Klebsiella pneumoniae, Staphylococcus, and Enterococcusaccount for some cases. Approximately half of all complicated UTIs are caused by multiple bacteria. In these complex infections, the etiological bacteria are different from those in simple UTIs; specifically, E. coli is less common, and Pseudomonas aeruginosa, Serratia, Staphylococcus, and Enterococcus are observed at higher frequencies.
The verification of pyuria (leukocyturia) and bacteriuria in a correctly collected urine sample is essential for making a diagnosis of a UTI. A diagnosis of significant pyuria is made when the WBC count in a urinary sediment examination is 5/HPF or higher. Meanwhile, bacteriuria is diagnosed by a quantitative culture. In case of midstream urine, significant bacteriuria is generally defined as a bacterial count of 104–105 CFU/mL or higher. A score of (1+) in a urinary sediment examination is equivalent to 104–105 CFU/mL. However, urinary sediment examinations are conducted using centrifuged urine specimens, whereas urine specimens are subjected to culture without prior centrifugation. Therefore, the number of bacteria in a urinary sediment examination depends on the specific gravity of the specimen and may not always agree with the culture result.
Fungi appear grayish white to pale green and Candida-like and are thus relatively easily differentiated. However, caution should be exercised as these cells sometimes appear similar to RBCs. In addition, segmented WBCs with apparent bare nuclei as a result of degradation may be mistaken for fungi. Fungi are more likely to appear during and after the administration of antibacterial agents as a result of alterations in intestinal bacterial flora. Fungi in the urinary tract often spontaneously disappear without any special treatment, and few patients require medical treatment. However, the risks of sepsis and multiorgan spreading of infection increase in patients with reduced defenses against infection, such as the elderly, those with diabetes, and those receiving immunosuppressive therapies.
Because fungi reside in the vagina, the detection of these cells in urine samples from women indicates a range of possibilities, from simple contamination to a UTI. As is the case for bacteria, a diagnosis of UTIs caused by fungi requires evidence of pyuria and funguria; a count of 104–105 CFU/mL or higher is generally considered to result in a diagnosis of significant funguria.
Protozoa found in urinary sediments generally comprise T. vaginalis. Although T. vaginalis is mainly found in women, it is also found in men and is often accompanied by the presence of squamous epithelial cells. These protozoan cells have a pear-like shape with longest and shortest diameters of 10–15 μm and 6–12 μm, respectively, as well as five flagella. Actively moving protozoa are easily confirmed, whereas inactive cells must be differentiated from WBCs, which have a similar appearance. The presence of a shiny, pale grayish-white and flagella assist with differentiation. Further, protozoa are slightly thicker than WBCs and exhibit size variability.
Other protozoa found in urine include plankton, a natural contaminant.
Schistosoma haematobium eggs are rarely found in urine. These eggs appear in urine in some patients affected by parasitic S. haematobium in blood vessels of the venous plexus near the bladder and anus, which mainly deposit eggs in vanules of the bladder wall. The eggs are characterized by a spindle shape with a blunt rounded end and a yellowish brown eggshell with no operculum. The longer and shorter diameters are 110–170 μm and 40–70 μm, respectively, and a thorn is present in the tail end. S. haematobium is distributed across Africa, the Middle East, and India. Because the eggs and surrounding tissue drop into the bladder, the main symptoms are hematuria and pain during urination. The bladder wall becomes increasingly hyperplastic and fibrous, and a high incidence of bladder cancer has been noted and linked to parasitic infection in endemic areas such as Egypt.
In addition, Strongyloides stercoralis can be found in urine. This organism is parasitic in the mucous membrane of the small intestine but can be found in the sputum, bronchial lavage fluid, cerebrospinal fluid, urine, pleural effusion, or ascites from patients with reduced immunity.
Other contaminating parasites found in urine include pinworm eggs.
Salts/crystals in urine mainly depend on consumed food and drink and salt metabolism in the body and comprise components that are filtered in the kidney and have precipitated in the urinary system or after urination in urine collection containers as a result of reduced solubility through a variety of physical and chemical actions (e.g., concentration, pH, temperature, or coexisting substances).
Salts/crystals include normal crystals, which are also seen in healthy individuals; abnormal crystals, which reflect pathological states; and drug crystals, which are derived from drugs taken by a patient or administered by a doctor. Oxalates, urates, and phosphates account for majority of the crystals frequently observed during routine practices. However, the differentiation of abnormal crystals, such as amino acid crystals and nucleic acid crystals, observed in patients with severe liver damage and congenital metabolic abnormalities is clinically important. Abnormal crystals include bilirubin, cholesterol, cystine, 2,8-dihydroxyadenine, tyrosine, and leucine. Drug crystals are often present in a crystal form that differs from the form of the original drug as most administered drugs are metabolized in the body and undergo structural changes.
Many salts/crystals exhibit unique morphological features and limited varieties depending on urine pH. Therefore, these crystals can be distinguished by a microscopic examination. However, for analogous components and abnormal crystals, further confirmation of solubility with acid or alkaline solutions and detailed analyses are necessary. (Figure 2.33)

Figure 2.33
Relationships of various salts/crystals with pH (sited from “General Survey Technical Textbook” (2012)3) p. 79 Fig. 4-43)
Normal crystals are generally considered to have a low clinical significance because they are also detected in healthy individuals. However, attention should still be paid to normal crystals as they might indicate the cause of urinary tract stones and calcium metabolic abnormalities.
These colorless, refractive crystals are observed in various shapes (e.g., regular octahedron, dumbbell-like, biscuit-like, and elliptical) and are often found in acidic urine, but they may also be observed in alkaline urine. These crystals are insoluble in acetic acid and slowly dissolve in hydrochloric acid. They may appear after consuming a large amount of foods containing abundant oxalic acid (e.g., oranges, tomatoes, spinach, and asparagus). The cause of calcium oxalate stones, which account for 80% of urinary tract stones, is unknown but is closely related to eating habits.
Urate crystals are colorless to yellowish brown crystals found in various shapes (e.g., whetstone-like, diamond, and pillar-like). Caution may be necessary as they sometimes appear similar to cystine crystals or cholesterol crystals. Urate crystals are found in association with aciduria and are dissolved by heating or with potassium hydroxide or aqueous ammonia.
Calcium phosphate crystals appear colorless to grayish-white and are thin and irregularly shaped (e.g., plate-like and pillar-like). These crystals are found in association with alkaluria, neutral urine, and mildly acidic urine and are dissolved in hydrochloric acid or acetic acid.
These colorless, refractive crystals appear in various shapes (e.g., coffin lid-like, envelope-like, and prism), are found in association with alkaluria and neutral urine, and dissolve in hydrochloric acid or acetic acid.
These spherical crystals present with brown thorns and are often found in alkaluria. They dissolve in hydrochloric acid, acetic acid, or potassium hydroxide.
*Ammonium acid urate crystals
These spherical crystals with brown thorns are morphologically similar to ammonium urate crystals and dissolve by heating and in potassium hydroxide. These crystals rapidly form stones in infants with infectious gastroenteritis (e.g., rotavirus gastroenteritis) or in situations of laxative abuse with a background of excessive dieting, and the number of cases of post-renal acute renal failure due to stones is increasing. Infrared spectroscopy is used for differentiation; however, these crystals, when identified in mildly acidic and strongly ketone body-positive urine, should be reported as suspected ammonium acid urate crystals.
These colorless non-crystalline granular, small-spherical, or biscuit-like crystals are found in alkaline and neutral urine and dissolve in hydrochloric acid and acetic acid while forming air bubbles.
Abnormal crystals are highly clinically significant and may directly lead to the diagnosis of congenital metabolic disorders, severe hepatic impairment, and other conditions. Therefore, abnormal crystals should always be reported, even if only a small number are detected.
These are yellowish brown, needle-like crystals. Bilirubin crystals are occasionally observed to adhere to WBCs or epithelial cells. These crystals are usually found in bilirubin-positive urine but are occasionally found in bilirubin-negative urine. They dissolve in chloroform and acetone. These crystals are associated with hepatobiliary diseases such as hepatitis and biliary obstruction.
These colorless, distorted rectangular plates are associated with nephrotic syndrome and chyluria. They dissolve in chloroform and ether.
These colorless, hexagonal plate crystals are found in cases of congenital cystinuria and Fanconi syndrome and are responsible for urinary tract stones. They are also observed in aciduria as the solubility of cystine decreases in acidic urine. These crystals dissolve in hydrochloric acid, potassium hydroxide, and aqueous ammonia.
These crystals are pale yellow to brown, radial circular/spherical crystals observed in cases of aciduria and are found in cases of urolithiasis associated with congenital adenine phosphoribosyltransferase deficiency. They appear similar to uric acid salts but are not dissolved by warming or in EDTA salt-containing saline. These crystals can be identified by infrared spectroscopy or X-ray diffraction.
These are colorless needle-like or tubular crystals with a radial extension and are reportedly observed under conditions of aciduria associated with severe hepatic parenchymal disorders. They are soluble in hydrochloric acid and potassium hydroxide.
These are pale yellow concentric or radial round crystals that infrequently appear in cases of severe hepatic parenchyma disorders and dissolve in hydrochloric acid and potassium hydroxide.
Granules formed from hemosiderin, a pigment in the body, are yellowish brown granules containing iron derived from hemoglobin. With S staining, hemosiderin granules are stained magenta, and it may be difficult to differentiate these from hemosiderin casts that incorporate granules or granular casts. Berlin blue staining is used to confirm hemosiderin granules.
In diseases that cause intravascular hemolysis, hemoglobin is released from disrupted RBCs and escapes glomerular filtration by mainly binding to haptoglobin; however, hemoglobin is filtered from glomeruli when its concentration exceeds the binding capacity of haptoglobin. Hemoglobin in the glomerular filtrate (primary urine) is partially reabsorbed through renal tubules and is converted to hemosiderin within cells. These hemosiderin-containing cells desquamate, are excreted in urine, and are observed as yellowish brown hemosiderin granules, hemosiderin-containing cells, or hemosiderin casts containing granules.
Hemosiderin granules are often found in conjunction with diseases that cause intravascular hemolysis, such as paroxysmal nocturnal hemoglobinuria, acute hemolytic anemia, and idiopathic portal hypertension, as well as after incompatible blood transfusion, and large-volume blood transfusion and in patients using artificial heart valves and with marching syndrome.
Contaminants found in urinary sediments include not only cellular components derived from the urinary tract but also contrast agents and lubrication oil used for diagnosis and treatment, as well as semen components (e.g., spermatozoa, gonadal secretions, corpora amylacea, and lecithin granules) in urine samples from men. Although contaminated semen components lack clinical significance, attention should be given as these can cause the false-positive detection of proteinuria.
Urine samples from women and infants are often contaminated by feces during urine collection. Although samples from men are generally not contaminated by feces, fecaluria may occur in association with a rectovesical fistula due to rectal cancer invasion to the bladder, which creates a passage between the bladder and the intestinal tract. Therefore, fecal contaminants should be carefully observed as their detection in men occasionally leads to the discovery of rectal cancer.
Urine cups intended for urine collection must be kept clean until use. Cups left near a bed may be contaminated by unexpected factors such as air-borne pollens, scales, and dead mites. Care should also be taken to avoid contamination by feces attached to a urine collection bag and fibers from paper diapers as these may yield misleading urinary sediment evaluation results.
VI Automated Urinary Sediment Examination: Concept of Urine Formed Element Analyzer Operation
Today, analyzers based on the new concept of urine formed element information have been introduced for a urinary sediment examination and have been implemented in the automation/streamlining of urine testing.
Urine formed element information is measured according to two major principles: the image processing method, in which elements are classified using captured urinary sediment images and image analysis systems, and the cytometry-based method. The benefits of urine formed element analysis include reduced labor, more rapid routine testing services, the provision of information with images of elements that can be saved using some systems to clinics, and educational usefulness. However, users should fully understand that this type of analysis differs from a urinary sediment examination in some aspects such as the accuracy of minor component detection and the limitations of a detailed classification. Therefore, along with establishing a verification system of the outputs with qualitative urinary examination results and previous values, clinicians should be consulted prior to an operation to determine what each clinical department requires from urinary sediment examinations as well as to explain the characteristics of urine formed element information and thus further the understanding.
The proposed JCCLS urinary sediment examination guidelines explicitly state that automated instruments used for a urinary sediment examination should be implemented with an adequate understanding of their characteristics. In other words, the guideline indicates that these instruments represent a new urine formed element information analysis, rather than mechanical automation of a standard urinary sediment examination.
In a manner of speaking, this is an automated form of microscopic element analysis; however, the challenge is to determine whether this method can be used to record clear images and accurately analyze elements. Currently, the detailed classification of epithelial cells and casts is limited with this method, although this limitation varies depending on the instrument model. In this case, technicians manually classify elements using saved images displayed on the screen; however, introductory points would address whether these images are satisfactory compared to images obtained under a microscope and whether a series of operational processes would constitute reasonable routine examinations.
This method aims to differentially stain the characteristics of elements (i.e., size, shape, and nuclei) using fluorescent dyes and to display and analyze these elements on scattergrams generated by measuring light scattering and fluorescence emission resulting from laser beam stimulation. This method is characterized by the clear analysis of the distribution of roughly classified present elements; the contents of these elements in non-centrifuged urine (counts/μL) can be measured quickly, although there are limitations in the detailed classification of elements other than RBCs and WBCs. However, microscopic examinations are essential for detailed classifications.
VII Quality Control
Because urinary sediment examination results significantly depend on the technical competence of the microscopy technician, each facility should implement training curricula and train technicians as part of their internal quality control. To conduct urinary sediment examinations, the microscopic examination skills of each technician should be evaluated and measures such as re-education should be taken when needed.
In addition, the person in charge of each microscopic examination should be designated, and this designation should be kept on record.
Internal quality control for a urinary sediment examination should include a patient information check at the time of the microscopic examination, inter-item check, and previous value check; ideally, these checks would be performed using an examination system. In addition, examination system-based methods would include positive rate/negative rate checks of various factors using results from daily examinations.
In one double-check method, identical samples are subjected to microscopic examinations by multiple technicians; this method uses daily specimens or fixed specimens for education. The double-check method can also be used to improve element discrimination abilities and thus has educational benefits. In recent years, web-based photo surveys have been offered, and it is important for individuals to participate in such surveys and thus improve their discrimination capabilities. The quality control of automated urine formed element analyzers, which have become increasingly common in recent years, can be done using methods similar to those used for automated biochemical analyzers.
The external quality assessment methods for a urinary sediment examination include participation in photo surveys offered by the JAMT, College of American Pathologists (CAP), and other organizations. Participation in external quality control surveys is an important step toward understanding inter-institutional differences. In addition, inter-technician differences and intra-institutional education effects can be improved using photo surveys and other components of quality control surveys.
www.djgkj.com
References
- 1) CLSI (formerly NCCLS): Urinalysis; Approved Guideline―Third Edition. CLSI document GP16-A3 (ISBN 1-56238-687-5). Clinical and Laboratory Standards Institute, Wayne, PA, 2009.
- 2) The European Confederation of Laboratory Medicine (ECLM): “European urinalysis guidelines,” 1–96, The Scandinavian Journal of Clinical & Laboratory Investigation Vol. 60, Taylor & Francis Group, UK, 2000.
- 3) Japanese Association of Medical Technologists: General Survey Technical Textbook, Tokyo, 2012.
评论
发表评论